Facet Dependence of the Oxygen Evolution Reaction on Co3O4, CoFe2O4, and Fe3O4 Epitaxial Film Electrocatalysts
Posting date on solidfuturism: May 23th 2024
Published date: may 8th 2024
Authors: Earl Matthew Davis, Arno Bergmann, Helmut Kuhlenbeck, and Beatriz Roldan Cuenya
DOI: 10.1021/jacs.3c13595
Abstract composer and editor: Seyed Amirhosein Mirsadri
Having accessible energy everywhere and at all times is of great importance to us. Creating an energy source or transporting it is an issue that needs attention. One of our ways to reach this sustainable energy that we can create anywhere and at any time is to reach energetic molecules through the electrochemical decomposition of water (Water splitting). In one direction in a half-cell electrochemical, oxygen is produced but the production of this oxidizing gas is accompanied by problems, one of which is its low yield. Therefore, scientists with the investment of the Haber Institute from the Max Planck Society in Berlin set out to study single crystal electrodes including Co and Fe with the design of single crystal electrodes. The reason for choosing this type of electrode was that they could precisely determine what happens to the physical and chemical nature of the material during the electrochemical reaction. Because in conventional electrochemical methods and with a lot of electrode, it is much harder to detect surface and depth reactions of metals and in single-sheet electrodes we can more easily and clearly understand the surface and internal electrode reactions.
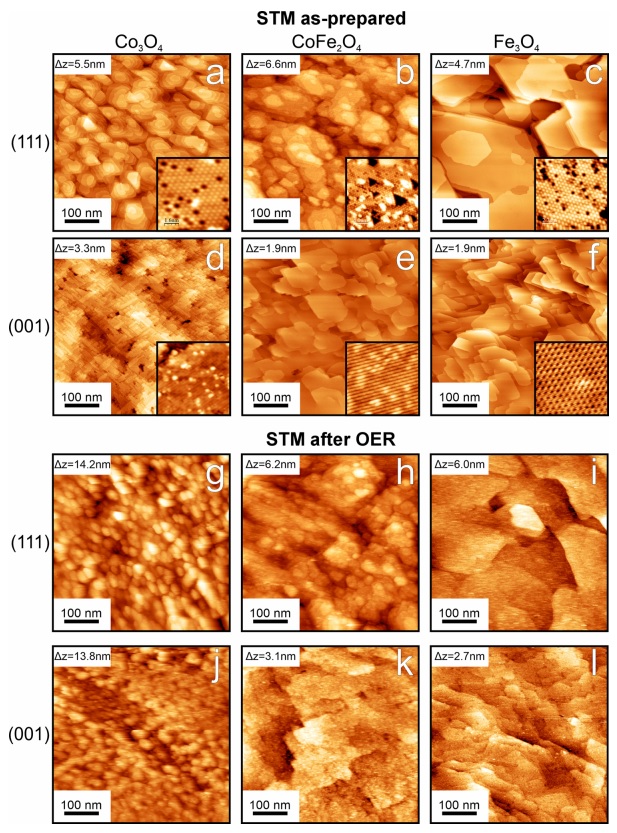
Therefore, three types of electrodes from cobalt oxide (Co3O4), iron oxide (Fe3O4) and iron and cobalt oxide (CoFe2O4) were synthesized with two types of sheets (001) and (111), a total of six electrodes were examined. The purpose of this experiment is to determine the reactivity, reaction rates on the electrode surface and the amount of oxide film production (including the type of metal oxide for retaining the produced oxygen) to be examined in order to determine the best type of sheet for this reaction. At this time, the surfaces of the metal electrodes were synthesized by a high-temperature synthesis method and examined by analytical methods. Since cobalt is known to be able to penetrate the iron oxide structure and settle itself, the mixed compound CoFe2O4 was first synthesized by Fe3O4 and cobalt atoms at high temperature penetrated this film to obtain a high metal oxide layer. In the meantime, it should be known that after the production of oxygen, what is created on the surface of the electrode can be examined for concentration. On the surface of the electrodes, oxyhydroxides are formed, the increase in the number of oxygen in them can help us in identifying the amount of absorbed oxygen.
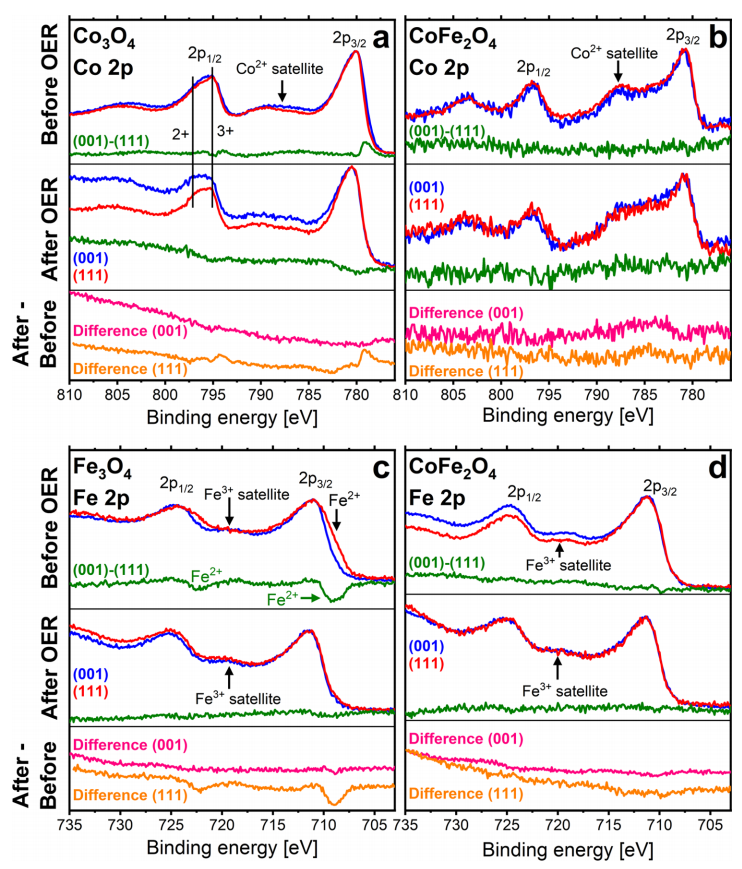
Therefore, by applying the current resulting from the chronoamperometry method over two hours of charging and discharging and with XPS analysis, they realized that on the surface of the electrode it could be compounds containing Co(OH) or CoOOH. To create these oxyhydroxides, studies showed that after applying charge and discharge or creating oxygen, the metal structure and its order are not disturbed by the oxyhydroxide layer, but this experiment showed that after this complete reaction, the surface of the electrode does not return to before and a little the surface of it changes compared to before and the reason is the presence of iron atom. For cobalt oxide films, it may be that the granular structure of the skin layers promotes further skin layer growth. Cobalt oxide can be granulated and this granularity may allow the electrolyte to still interact with the metal oxide of the underlying layer when there is already a layer of hydroxylated metal, thus promoting its further growth. The skin layers on the films that contain iron are smoother.
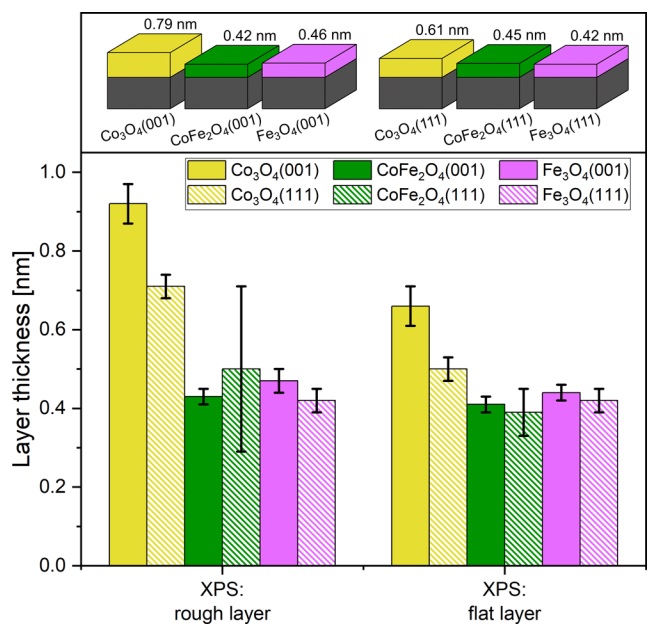
Therefore, the role of iron could also be that it leads to smoother and more closed layers, better protecting the underlying oxide from interaction with the electrolyte. XPS data for oxygen atoms with S1 orbital in cobalt oxide films, even if the samples are only under ultra-vacuum (UHV) conditions, the absence of iron makes the layers more sensitive to hydroxylation, which is somewhat in line with the observation of thicker skin layers after oxygen production reaction on cobalt oxides. OER reactions are facet-dependent. This may be traced back to the different facet structures, with the (001) facets exposing four and five-fold coordinated metal ions while the (111) facets expose only three-fold ones. The surface structures of the underlying oxide affect the structures of the thin skin layers which are directly involved in the reaction. For Co3O4, the (001) facet is clearly more reactive, while for the other oxides, the (111) facet is more active.